From designer microbes to stem cells, U of T researchers are investigating new strategies to treat bowel disease
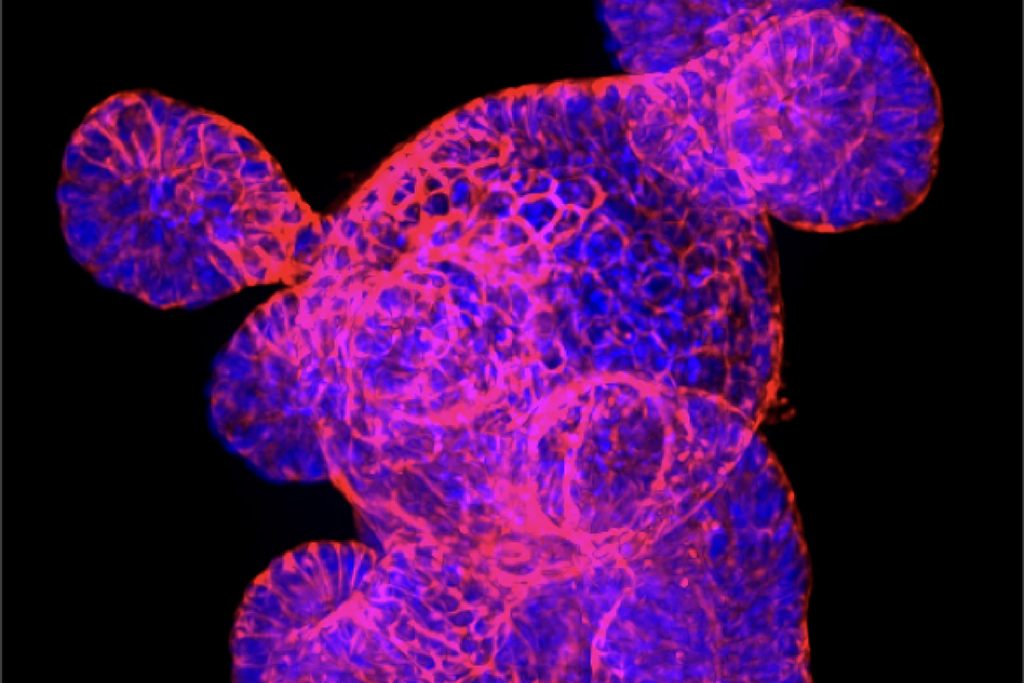
The University of Toronto’s David McMillen and his research team are creating a new life form to ward off disease – a bespoke, gut-dwelling microbe engineered to release drugs when needed.
“Our ideal outcome would be to be able to deliver a therapeutic into the gut with a programmable bacterium, which you could use for multiple diseases,” says McMillen, an associate professor in the department of chemical and physical sciences at the University of Toronto Mississauga and leader of a Medicine by Design team looking to develop regenerative medicine therapies for intestinal diseases.
This new type of approach is one of several interdisciplinary projects funded by the regenerative medicine initiative with the aim of harnessing intestinal stem cells – cells that can self-renew and replace damaged cells in the gut – to boost the body’s natural healing ability and perhaps even grow replacement tissue in a dish.
Other efforts are led by Jeffrey Wrana, a senior investigator at Sinai Health System’s Lunenfeld-Tanenbaum Research Institute (LTRI) and a professor in U of T’s department of molecular genetics, and Ulrich Tepass, a professor in the department of cell and systems biology, who are investigating how different cells contribute to gut development and maintenance.
The three team projects are among 19 from across U of T and its affiliated hospitals that Medicine by Design is funding to accelerate stem cell discoveries and translate them into new treatments. Medicine by Design is made possible thanks in part to a $114-million grant from the federal government’s Canada First Research Excellence Fund – the largest single research award in U of T’s history.
Germs to the rescue
As many as 100 billion cells are thought to be shed every day from the intestine as part of wear and tear brought about by food digestion. The lost cells are quickly replaced, thanks to stem cells that dot the entire length of the intestine and are tasked with repairing tissue damage throughout life. But when this cell shedding and replacement process goes awry, it can lead to a host of diseases, from inflammatory bowel disease (IBD) to cancer.
If, for example, the cells of the intestinal lining are not replaced quickly enough, the gut can become “leaky” and unable to properly absorb food, clear waste and most importantly, prevent microbes from entering the body. This triggers inflammation, as seen in IBD, an umbrella term for Crohn’s disease and ulcerative colitis, both of which negatively impact quality of life.
With one in 150 Canadians living with Crohn’s or colitis, Canada has one of the highest incidences of IBD in the world, with economic costs estimated at $2.8 billion per year, according to the 2012 report by the Crohn’s and Colitis Foundation of Canada. Among Canadian children under the age of five, the incidence of IBD is rising at an alarming rate, according to a study published last year.
While it is not clear what is behind the rise in IBD, it is thought that the microbiome – the gut’s resident bacteria – could have something to do with it. Dubbed by experts as the “neglected organ,” the microbiome is increasingly viewed as crucial for human health, especially for the proper development of the immune system.
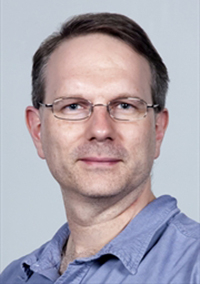
It’s fitting, then, that a Medicine by Design team is looking to fix the gut lining by means of an engineered gut microbe. Led by McMillen (pictured left), the team will insert genes into a bacterium that will allow it to sense and respond to inflammation in the gut in such a way that it can treat – and possibly even cure – IBD.
The project is rooted in the link between the microbiome and Crohn’s disease, previously discovered by McMillen’s collaborator Dana Philpott, a professor in the department of immunology. Philpott’s team found that gut bacteria secrete a product called muramyl dipeptide (MDP), which binds to a receptor called NOD2 that resides inside the stem cells. A recent study showed that MDP acts via NOD2 to spur intestinal stem cells to proliferate and generate new cells for lining repair.
Current treatments include injections of steroids and other anti-inflammatory drugs that cause whole-body immunosuppression, which can be harmful. But these “band-aid sort of approaches” don’t address the root of the problem and merely reduce the symptoms, says Philpott. By making a gut bacterium that can ramp up production of MDP during inflammation, “it should be possible to stimulate the human receptor to the right level and promote healing of the cells that are hardest hit during Crohn’s disease,” Philpott says.
To do this, the researchers will insert a synthetic genetic circuit inside a bacterium that will allow it to detect inflammatory molecules in the gut and use this as a trigger to release MDP for tissue repair.
Creating disease-fighting bacteria is at the cutting edge of synthetic biology, a field that strives to create cells with new and desired properties. “The main advantage bacteria have over drugs is that they can also sense disease,” says McMillen.
Microbiome engineering is particularly appealing not only because bacterial genomes are small and fairly easy to tweak, but also because getting regulatory approval for any such therapy should be easier than for engineered human cells.
“Of all the things you could engineer, the microbiome is probably one of the safest places where you could start applying synthetic biology,” says Keith Pardee, assistant professor at the Leslie Dan Faculty of Pharmacy, who is also on the team. “You’re not modifying the patient, you are modifying the bacteria that will eventually wash out.”
The team is taking advantage of the fact that gut microbes change their gene expression – and ultimately the molecules they release – in response to inflammation. Working with mice, their plan is to identify these molecules and turn them into universal inflammation sensors. As high-tech as it sounds, the daily grind of experiments is far from glamorous. It involves collecting mouse droppings to get the excreted bacteria, which are then mashed up and analyzed by DNA sequencing to find the genes that turn on in response to IBD.
This part of the project is being carried out in collaboration with John Parkinson, a senior scientist at the Hospital for Sick Children (SickKids) and a professor in U of T’s department of molecular genetics, who is an expert in computational large-scale data analysis. Also on the team are Tae-Hee Kim, a scientist at SickKids and assistant professor in the department of molecular genetics, who is using a “gut on a chip” platform to study intestinal stem cells in more detail, and Radhakrishnan Mahadevan, a professor at U of T’s department of chemical engineering and applied chemistry, who is engineering bacteria to produce high levels of the MDP molecule.
Another possible approach the team is investigating involves dampening inflammation locally and letting the intestine recover on its own. In this case, an engineered bacterium would release anti-inflammatory drugs only at the site of inflammation, which is beneficial in preventing all-body immunosuppression.
And unlike most cutting-edge medicines, the therapeutic bacteria need not cost a fortune.
“What we’re making is self-replicating drugs that could treat patients essentially for free,” says Pardee. “Everyone can grow a bug.”
If all goes well, McMillen thinks that an IBD-sensing bacterium is within reach in three years’ time.
“This is the most exciting project of my career,” says McMillen. “Thanks to Medicine by Design, we have a shared goal among a group of researchers with a wide range of interests and this is catalyzing interactions and ideas we would not have had otherwise.”

Towards organ replacement and repair
Using stem cells to grow replacement organs from scratch – or to boost the body’s natural healing processes at will – are long-standing goals in regenerative medicine. But before these goals can be realized, researchers first need to get a clearer understanding of how different types of cells work together to drive organ growth and maintenance.
A few years ago, it became possible to grow three-dimensional balls of intestinal tissue from stem cells in a dish, also known as intestinal organoids or “mini-guts,” which allow researchers to examine the diverse roles different types of cells play in bowel health and disease.
Wrana and his team are at the forefront of organoid research. With Medicine by Design funding, they are now developing new imaging technologies that, combined with genome editing and mathematical modelling, will reveal new insights into how individual cells contribute to intestinal repair.
“These discoveries can be applied to making the growth of human replacement tissues more efficient, or by illuminating how to get replacement tissue to functionally integrate into the recipient organ,” Wrana says. “This research will also enable the design of therapies aimed at awakening dormant regenerative programs in damaged organs, thereby stimulating our own tissue to repair itself.”
In addition to John Parkinson, team members include Laurence Pelletier and Daniel Durocher, both senior investigators at LTRI and professors in the department of molecular genetics; Daniel Schramek, an investigator at LTRI and an assistant professor in the department of molecular genomics; Sevan Hopyan, a senior scientist at SickKids and an associate professor in the department of surgery; Liliana Attisano, a professor in the department of biochemistry and in the Donnelly Centre for Cellular and Biomolecular Research; Christopher Yip, a professor at the Institute of Biomaterials & Biomedical Engineering (IBBME); and Luca Scardovi, an associate professor in the Edward S. Rogers Sr. Department of Electrical & Computer Engineering.
Getting the right size
Before researchers can grow replacement organs in the lab, they’ll need a way to control their size. Organs that are too big or too small would be unsuitable for transplant, while any unchecked growth could potentially lead to cancer.
How organ size is controlled is what Tepass’s team is trying to find out.
For the intestine, the key again lies in the lining, which harbours stem cells. It’s now clear that cells that make up the lining do much more than just separate the intestine from other tissue. For example, as the intestine become distorted during food digestion, the cells in the lining sense and respond to mechanical forces by changing which genes are switched on. And when it comes to stem cells, stretching makes them proliferate more.
Being able to control this mechanism of cell proliferation could help regulate organ size in culture. “If the organ grows too fast, or becomes too big, you could use a drug that reduces mechano-sensitivity to slow down growth,” says Tepass, adding that, while this research is still in early stages, one day such approaches could find a place in the clinic.
Tepass is searching for molecular players that link mechanical stress to changes in gene expression. But he is not looking for answers inside the gut. Instead, his team is studying the wings of fruit flies. The wing is composed of the same kind of epithelial cell sheet that forms the gut lining and envelops many internal organs. The study of fly wings helped researchers discover numerous genes that control organ size, which were subsequently also shown to have the same function in humans. Working with flies allows researchers to investigate complex cellular behaviour in the context of a whole animal, which cannot be readily replicated in cultured cells or organoids – and at a fraction of the cost and time it would take to do the same in larger animals such as mice.
“The process of going from stem cells to a functional organ is still largely a black box, something we don’t know very well,” Tepass says. “To control the overall size of the organ is a challenging biological question and we are using a simple organism to find key molecular players that orchestrate how big an organ gets.”
Working with Helen McNeill, associate investigator at LTRI, and Anne-Claude Gingras, senior investigator at LTRI and professors in the department of molecular genetics, the team is identifying new genes and proteins that control growth. Also on the team is Jüri Reimand, principal investigator at the Ontario Institute for Cancer Research and an assistant professor in the department of medical biophysics, who is helping with large-scale computational data analysis. To see how these molecules are distributed in cells, Tepass has teamed up with Yip at IBBME and Rodrigo Fernandez-Gonzalez, an associate professor at IBBME and a member of the translational biology and engineering program at the Ted Rogers Centre for Heart Research, who are experts in combinatorial microscopy, live tissue imaging and quantitative image analysis.
Tepass emphasizes that “support from Medicine by Design has been essential to bringing together researchers from different fields to refocus their efforts on the question of controlling organ size. On a fundamental level, the understanding gained here is expected to guide rational approaches to organ regeneration and repair in the clinic.”